The alchemists of Ludwigshafen
Society’s fatal dependence on artificial fertiliser has its roots in the industrial revolution and the western world’s search for food security. Today we are on the brink of another breakthrough in human nutrition: but as history shows, new food technologies have unforeseen consequences.
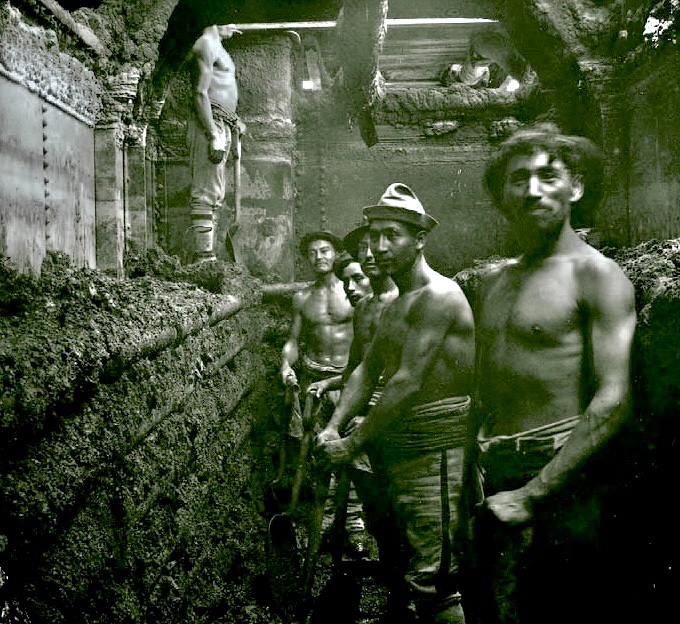
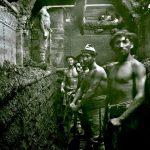
Food comes first in the order of humanity’s needs. Then come not only morals (Brecht), but also all the subtleties of advanced gastronomy. From this simple perspective, food is fuel: the fats, proteins and carbohydrates loaded with chemical energy that our metabolism converts into heat and movement, or into new energy sources in the body’s cells.
But larks don’t land in our mouths ready-cooked, as the author of the 14th-century satirical poem The Land of Cockaygne imagined it. Karl Marx once defined labour as the metabolism of society, through which nature is converted into energy. Producing, gathering, storing and processing food has been mankind’s core activity for most of its existence. If the energy consumed in work derives from the food we collect and produce, then human beings, like other animals, operate within a bodily or somatic energy regime. From this perspective, a labour process that uses up more energy than it provides is unsustainable.
Mankind the cultivator
When hunter-gatherers became farmers, they domesticated the function of some plants as converters of solar radiation into chemical energy. But this did not liberate humans from the somatic energy regime. Until then, it had mainly been muscle power that had chopped, ploughed and harvested. Now, however, a bottleneck appeared in humanity’s balance sheet with nature. To be able to grow and form cells, animals and plants need minerals and trace elements. But intensive and continuous cultivation disrupts the cycle of minerals and trace elements between stationary plants and the humus layers they grow out of and decompose into. When plants are repeatedly consumed away from where they are grown, the soil is depleted of trace elements. Nitrogen, phosphorus and potassium are leached from the soil and must somehow be returned.
Over millennia, and without deeper knowledge of the chemical processes involved, people cultivating the soil have developed methods to maintain this balance. Keeping the land fallow for longer or shorter periods is probably the oldest one. In riverine cultures and flooded field farming, the water carries with it large amounts of nutrient-rich sludge. Grazing animals collect nitrogen-rich feed from surrounding areas, producing excrement that farmers can use as fertiliser, sometimes along with their own. In a complex symbiosis with certain soil bacteria, legumes such as peas and beans capture and fixate part of the abundant nitrogen in the earth’s atmosphere.
But whenever farming people have found new ways to intensify the absorption of energy by cultivated land, they have been confronted with the challenge of returning trace elements to the soil. This was the case in 19th century Europe after the Industrial Revolution. With technology to convert heat into motion via steam and internal combustion engines, humanity entered an out-of-body, or exosomatic energy regime. Since then, most of the energy we consume has been independent of our biological metabolism. This transformation was followed by a few centuries of unprecedented population growth and urbanisation. Rising industrial production was one reason for this demographic transformation; but for it to be possible, food production also had to increase.
For classical economists such as Thomas Malthus and David Ricardo, food production appeared as the most dismal limitation on industrial transformation. Even if it were possible to imagine that machines and fossil fuels would take over from physical human labour in tilling and harvesting, arable land was still a truly finite resource. Experience taught that the more mechanical labour one put into the soil, the lower the marginal yield. Ricardo put his hope in free trade. If the population grew in the industrialised world, then the fields in the global periphery could feed it. But it was a temporary salvation at best. In addition to the strategic risk of people’s livelihood becoming dependent on long-distance trade, the world’s total arable land was still finite.
An acrid scent of guano
One way to deal with the constraint was to add fertilisers to the flow of raw materials and food that the industrialised regions received from the global periphery. But embedded in this flow was also a significant amount of good old-fashioned somatic energy, derived from onerous physical labour. Paradoxically, the nutrients that flowed into the western world’s bountiful lands came from some of the least fertile places on the planet.
An early solution to the nitrogen shortage was found on the three Chincha Islands off the coast of Peru. Over millennia, their cliffs had grown many times larger through the accumulation of fossilised guano from the cormorants and other birds that nested on the islands and fed on the extremely fish-rich waters. The area’s relatively dry climate also helped preserve nitrogen content in the guano. Between 1840 and 1870, hundreds of merchant ships carried this fetid cargo from the Pacific Ocean to European grain fields every year.
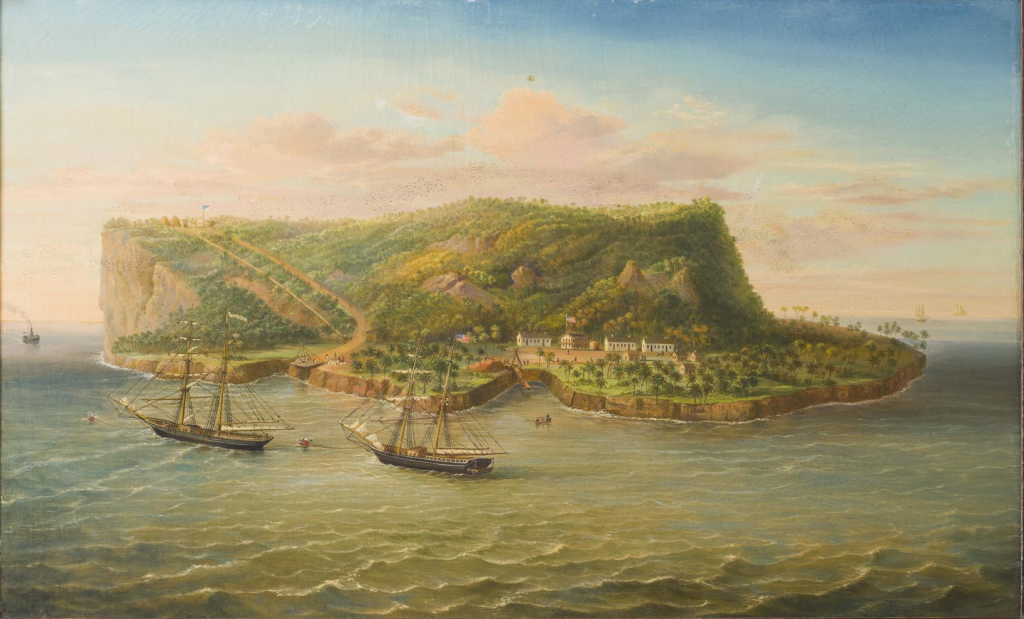
An unsigned painting of Navassa Island, circa 1870. The brig Romance is anchored in front of the company settlement near the shore with its guano mining operations visible up the hill. Source: Wikimedia Commons
Working conditions were abominable. Most of the guano mining in the Chincha Islands was carried out by indentured Chinese labourers. They were recruited on five-year contracts that many of them never survived. The extremely high death rate can be attributed in part to harsh working conditions and meagre food rations, but it was mainly due to the lung damage workers suffered when they were forced to inhale corrosive ammonia gases.Clark, B. & Foster, J. G., ‘Ecological Imperialism and the Global Metabolic Rift. Unequal Exchange and the Guano/Nitrates Trade’, in International Journal of Comparative Sociology Vol 50 (3-4) 2009, 311–334.
Enslaved Africans and Polynesians were also used. In 1862, for example, a third of the remaining indigenous population of Easter Island was abducted and taken to the guano quarries. About 90 per cent of these approximately 1,000 people died on the islands before international protests and pressure forced a repatriation of the last, badly debilitated survivors in the early 1870s.Maude, H. E., Slavers in Paradise: The Peruvian Slave Trade in Polynesia, 1862–1864, Stanford University Press 1981.
The guano trade was so lucrative that Spain, which had lost all of its American colonies except Cuba and Puerto Rico during the Napoleonic wars of the early 19th century, occupied the islands in the so-called Chincha War of 1864–66. When the islands were returned to Peru in 1871, the mountains of white guano had been virtually obliterated.
A search for new sources of guano began. The United States enacted the Guano Island Act in 1856, which granted American companies the right to exploit and annex any guano islands they could find on the world’s oceans, as long as they were uninhabited and not already exploited. At the end of the American Civil War, this law formed the basis for the emergence of a vast empire consisting of more than 60 annexed islands in the Pacific, Caribbean and Atlantic. It was the emerging superpower’s first territorial expansion beyond the American continent.
However, most of these new islands had smaller and poorer supplies than the Chincha Islands. They were relatively rich in phosphorus, but the nitrogen content was often leached by rain and wind. Moreover, many were difficult to access. It was obvious that the earth’s guano resources would soon be exhausted unless new sources of nitrogen could be found.
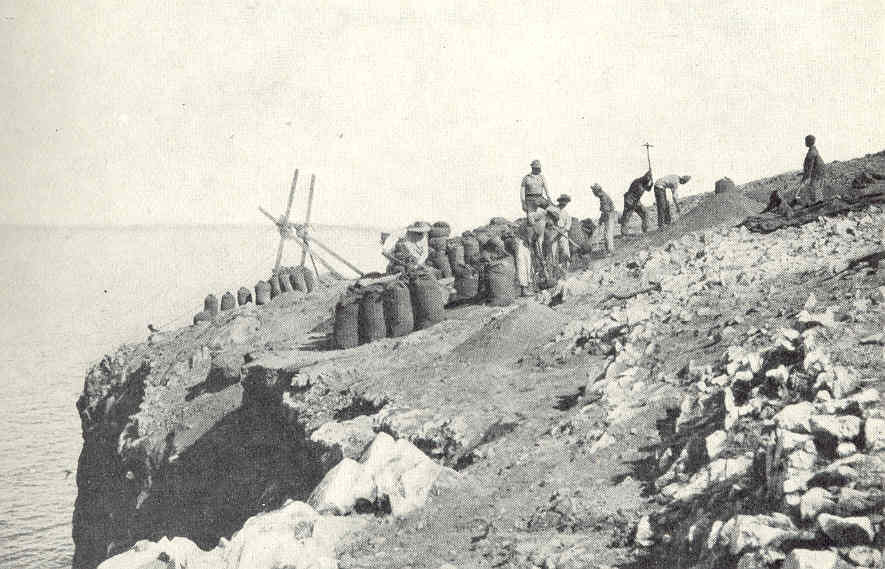
Sacking guano, Ballestas Islands (1910). Source: Wikimedia Commons
Finding nourishment in the desert
The solution was discovered not far south of the Chincha Islands, in the Atacama Desert, which at that time was shared by Bolivia, Chile and Peru. This plateau on the slopes of the Andes had once been a seabed and had accumulated large deposits of dead sea creatures. Under the area’s extreme climatological conditions, these organic residues had oxidised into a nitrogen-rich sodium nitrate. This fertiliser was presented to the farmers of the western world under the name Chile saltpetre. Chile, whose exports were favoured by British investors, acquired sovereignty over these deposits in the so-called saltpetre war of 1879–84.
Between 1880 and 1913, saltpetre mining in the Atacama grew into an industry that employed around 300,000 workers and exported up to four million tonnes annually. These nitrogen supplies were also dependent on heavy manual labour.Whitbeck, R. H., ‘Chilean Nitrate and the Nitrogen Revolution’ Economic Geography Vol. 7 (3), 1931, 273–283.
But although the supply of Chilean nitrate seemed inexhaustible, dependence on imported fertiliser was a concern for many European nations. The highly concentrated fossil nitrogen was found in a few remote locations. Whoever controlled the source and distribution of the substance controlled the food security of Europeans. The fact that western Europe’s food supply became increasingly dependent on imported grain from North America, Australia and Russia during the 50-year period prior to the First World War only increased this concern.O’Rourke, K. H., ‘The European Grain Invasion, 1870–1913’ in: The Journal of Economic History, Vol 57 (4), 1997.
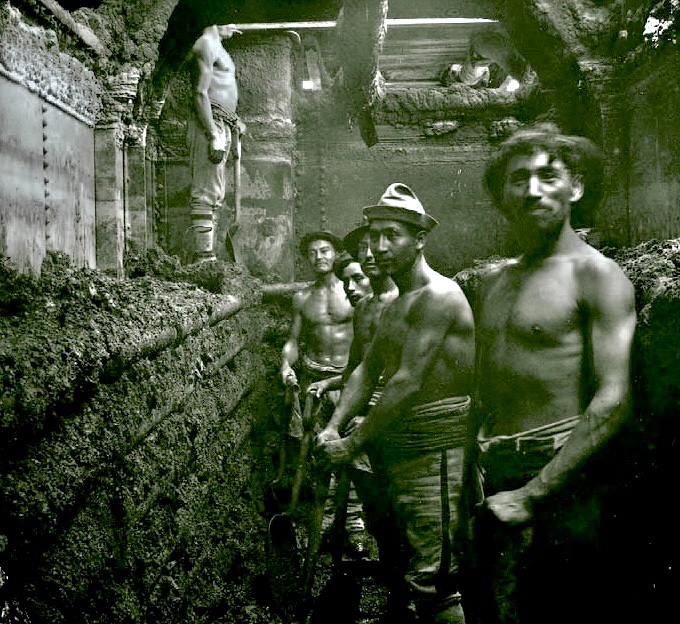
Chliean saltpetre workers, earlly 20th century. Source: Wikimedia Commons
A German dilemma
The threat was particularly acute in the continent’s fastest growing industrialised nation, the newly unified German Empire. More than anywhere else, German industrialisation in the final decades of the 19th century was a national and military strategy, directed by the ‘Iron Chancellor’ Otto von Bismarck.
Before the Napoleonic Wars, eastern Germany had supplied a significant amount of surplus grain to western Europe. As a supplier of food and raw materials, however, Germany had never thought it could establish the military muscle required to challenge the British and French in the long term. In the development and integration project that comprised German reunification, this changed. The focus shifted to matching and surpassing the industrialised and empire-building neighbouring countries industrially, technologically and colonially.
Rapid industrial development appeared to be both a means and an end. Germany became a world leader in what is usually described as a second industrial revolution, characterised by heavy metallurgy, weapons technologies, the chemicals industry, electrical engineering and internal combustion engines.
Food production also had a role to play in the development plan, but it had not grown at the same rate as industrial production and the population, which from approx. 35 million in 1850 had grown to around 65 million in 1913. Instead of a cereal surplus, there was now an import dependency of up to 25 per cent of consumption. At the same time, the remaining domestic production had become increasingly reliant on imported fertilisers. Between 1900 and 1912, imports of Chile saltpetre had grown from 350,000 to 900,000 tonnes. That year, Germany imported nearly 38 per cent of all the Chile saltpetre produced.1914 – 1918 online: International Encyclopedia of the First World War. Keywords: ‘Nitrate’, https://encyclopedia.1914-1918-online.net/article/nitrate 2023-02-24
Enter the chemists
‘Whoever could make two ears of corn or two blades of grass to grow upon a spot of ground where only one grew before, would deserve better of mankind, and do more essential service to his country than the whole race of politicians put together.’
Jonathan Swift, Gulliver’s Travels (Voyage to Brobdingnag), 1726
The dilemma became apparent with the emergence of rival blocs around the turn of the twentieth century. Germany and the Central Powers were trapped on the continent. Their adversaries controlled the world’s oceans and maritime trade. In the event of a conflict, it seemed unlikely that Germany’s military and industrial capacity would be able to cope if its soldiers and civilians began to starve.
Compounding the dilemma was that Chile saltpetre and guano were required not only for their life-giving function, but also as strategically indispensable raw materials in the industrial production of ammunition and modern explosives. These concerns would prove justified when war broke out. Germany was completely cut off from fertiliser imports between 1915 and 1918.Ibid
In Germany the search began for a technical solution. The second half of the 19th century and the early years of the 20th century was the age not just of electricity and the internal combustion engine, but perhaps even more so of chemistry. The science was at the time being revolutionised by breakthroughs in organic synthesis, catalysis research and electrochemistry.
For the first time in human history, molecules that did not exist in nature could be produced in laboratories and in large-scale industrial processes, while substances that also existed naturally could be produced synthetically. Elements could move between solid, liquid and gaseous aggregations (at constant temperatures) when the molecules they were part of were rearranged.
This created a breathtaking opportunity. In the 1840s, the British chemist John Lowes had developed a method for extracting super-phosphates from phosphorus ore using sulphuric acid; potassium could now also be extracted relatively abundantly from both ores and organic sources. This meant that nitrogen as a trace element had become even scarcer as a component needed to secure a strategic local supply of fertilizers.
However, the deficiency applied only to the nitrogen compounds found in solid form in the humus layer and the earth’s crust. As a gas, the nitrogen volume constitutes about seventy-eight per cent of the Earth’s atmosphere. If an economically viable way could be found to fixate a tiny fraction of this gas into a solid that could be mixed with the arable soil, it could be the magic formula that freed Germany from the crippling threat of starvation in the event of a major conflict. The fact that it would also provide the empire with brand new resources to produce ammunition would be no small bonus.
To anyone who looked up for a moment from the national chauvinism of geopolitics, the process promised to liberate societies once and for all one from the most tangible constraint on human sustenance.
Around the turn of the century, several methods were developed to fixate atmospheric nitrogen into liquids and solids. However, the earliest experiments were far too energy-intensive to be developed on an industrial scale.
It was Fritz Haber, perhaps the most acclaimed (and infamousHaber was born in 1868 as the son of a Jewish paint merchant in Breslau (Wrocław) in what is now Polish Silesia. He became an ardent German nationalist who wanted to put his science at the service of his country. Besides the Haber-Bosch synthesis, he is probably best known as the mastermind of German chemical warfare during World War I, with the development of weaponised gases such as chlorine and mustard gas. He also patented a prussic acid pesticide under the trade name Zyklon-B. However, he can hardly be blamed for its use in the gas chambers during the Holocaust. When the Nazis came to power in 1933, he was stripped of all his positions because of his Jewish background. He died in exile in Switzerland in 1934. Lena Einhorn has written a fine documentary novel about Haber’s life up to 1915 and about the moral conflict that the First World War caused between him and his wife Clara Immerwahr (also a brilliant chemist), Geniet från Breslau [The Genius from Breslau], Natur & Kultur, 2018.
) of the many brilliant German chemists of the time, who developed an economically viable method for chemical nitrogen fixation using ammonia synthesis. Basically, the method involves synthesising nitrogen and hydrogen under high pressure and high temperature to form ammonia, with oxidised iron as a catalyst. To develop the method for industry, Haber began a collaboration with the chemical engineer Carl Bosch at Germany’s largest chemical group: BASF in Ludwigshafen. The method was named the Haber-Bosch synthesis. It was a collaboration that gave BASF a monopoly on synthetic ammonia to produce fertilisers and ammunition. The factory in Ludwigshafen was completed in 1913, the year before the Great War broke out. It was supplemented in 1916 by the giant Leunawerke chemicals plant in Saxony-Anhalt, where eastern Germany’s rich lignite reserves could satisfy the high energy demands of ammonia production.The innovation came too late for Germany’s wartime supply chain. Hunger, particularly in urban areas of Germany and Austria, was a major factor in the surrender. To an even greater degree, it came to be used as an argument for the development of a higher degree of domestic self-sufficiency during the interwar period. 1914 – 1918 online, Keywords: ‘Food and nutrition’.
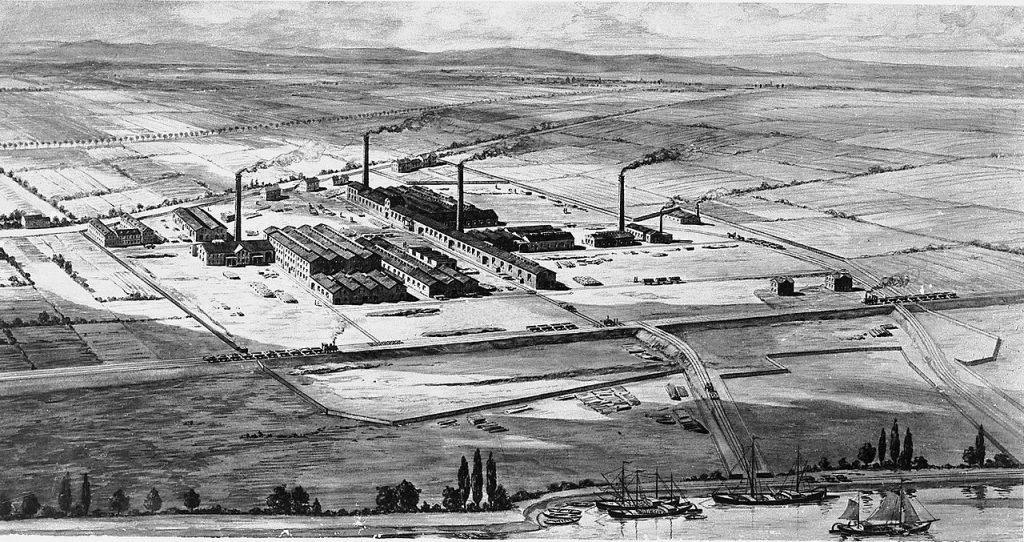
BASF factory in Ludwigshafen, 1865. Source: Wikimedia Commons
When Haber was awarded the Nobel Prize in Chemistry shortly after the end of the war in 1918, he was celebrated in the award citation as the magician who saved the world from hunger by making bread out of thin air. Estimates vary regarding how the Haber-Bosch synthesis process impacted on the global supply capacity, but they often fall somewhere between two and four billion mouths fed.Smil, V., Enriching the Earth. Fritz Haber, Carl Bosch and the Transformation of World Food Production, MIT Press 2001, 156ff.
The era of artificial fertiliser
It was only after 1945 that artificial fertilisers based on fixed nitrogen really took off globally, increasing from a global consumption of about 4 million tonnes in 1940 to 40 million tonnes in around 1960 and 150 million tonnes in the early 1990s.McNeill, J. R., Någonting är nytt under solen. Nittonhundratalets miljöhistoria [Something new under the sun. Twentieth-century environmental history], SNS publication 2003, 45.
The expansion was linked to growing free trade and the fact that patents and processes became more available outside Germany. But the production of cheap fertilisers was determined to an even greater degree by another basic condition: access to cheap energy. The molecules are charged with chemical energy and heat energy is lost in the conversion process. This is why the Leunawerke was placed next to the East German lignite fields. If the Haber-Bosch process makes bread from thin air, it also makes food from oil and coal. But only for as long as fossil fuels dominate our energy supply. The energy-intensive production of fertilisers in combination with the fact they give off nitrous oxide when spread on the fields has resulted in their share being calculated at approximately one fortieth of global greenhouse gas emissions.Ledo, A., Menegat, S. & Tirado, R., ‘Greenhouse gas emissions from global production and use of nitrogen synthetic fertilisers in agriculture’, in Nature Scientific reports, https://www.nature.com/articles/s41598-022-18773-w 2023-02-24
There is also an increasing correlation between food and energy prices from the post-war period onwards. Artificial fertilizers have clearly been central to this. For example, when the Russian gas tap was turned off in the autumn of 2022, fertiliser prices sky-rocketed, prompting many analysts to warn of a looming global food crisis.Bogmans, C., Pescatori, A. & Prifti, E.. ‘Global Food Prices to Remain Elevated Amid War, Costly Energy, La Niña’ IMF Blog: Commodities 9, December 2022, https://www.imf.org/en/Blogs/Articles/2022/12/09/global-food-prices-to-remain-elevated-amid-war-costly-energy-la-nina 24/02/2023
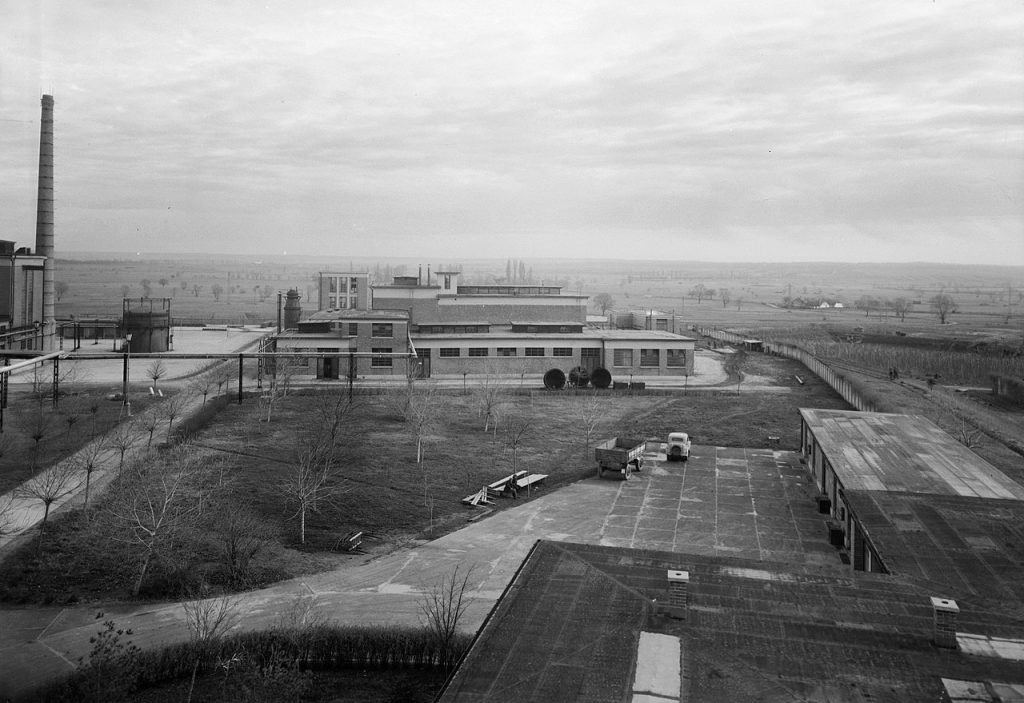
Péti Nitrogén Műtrágyagyár Rt. Photo: FORTEPAN / Veszprém Megyei Levéltár/Kozelka Tivadar / Source: Wikimedia Commons
Growing supply and falling relative prices for fertilisers have also had longer-term consequences. Fertiliser became so cheap that users would rather use too much than too little. It was no longer just a matter of restoring the balance of trace elements in the soil, but of increasing their concentration. The consequences are well known. The surplus was leached from the fields. The result has been eutrophication and disturbed oxygen balances in waterways and coastal seas. Only in recent years have techniques been developed that focus on ensuring that fertilisers are confined to the target area.
The new abundance of cheap fixed nitrogen has also had other consequences. The availability of artificial fertilisers changed the global food chain. Varieties of maize, wheat and rice requiring extra high nitrogen levels have spread at the expense of other crops. Today, two-thirds of the world’s cereal consumption comes from these three plants in a decreasing number of heavily bred and sometimes genetically engineered varieties. Even soybeans, which have become the dominant feed crop for the world’s rapidly growing meat industry, have proven extremely dependent on high doses of artificial fertilisers.Smil, V. Feeding the World. A Challenge for the Twenty-First Century, MIT Press 2000, 250ff.
What’s for dinner?
‘Your mother can’t produce food out of thin air,’ said Hermione. ‘No one can. Food is the first of the five principal exceptions to Gamp’s law of elemental transfiguration.’
J.K. Rowling, Harry Potter and the Deathly Hallows, 2007
One of Fritz Haber’s many grand scientific projects was his attempt to extract gold from seawater in order pay off German war debts after the First World War.Smil, V., Enriching the Earth. 228ff. It was the Swedish chemist Svante Arrhenius’ calculations that there was a gold content in seawater of about 2 milligrams per tonne that formed the basis of Haber’s experiments.
With historical distance, even more successful scientific breakthroughs sometimes recall the discovery of fairy-tale treasure at the end of the rainbow. At the same time, advances in knowledge have often generated more complex conflicts and limitations than those they overcame.
There can be no doubt that the process has proven crucial to sustaining humanity. However, our dependence on artificial fertilisers is the consequence of a choice. Storm clouds loom over the path we have chosen; reliance on cheap energy, increasing monoculture in world agriculture, eutrophication and accelerating oxygen depletion in our oceans and rivers, chemical imbalance in the humus layer, a looming shortage of phosphorus and other complementary trace elements, to name just a few.
New biochemical processes, both for fuelling our bodies with chemical energy and for catering to our culinary desires, are now appearing around the corner. Large-scale breeding of protein-rich larvae and industrial synthesizing of food from vegetable and animal stem cells are becoming increasingly realistic possibilities. Our knowledge of these choices is expanding rapidly. Yet we probably know far less than we need to about what such fundamental changes might mean for humanity.